Research
1. Catalytic valorization of woody biomass
Cellulose, the main component of woody biomass, is the most abundant biomass and is an inedible component that does not compete with food. We have succeeded in hydrolyzing cellulose using carbon as a solid catalyst to synthesize glucose. We are also investigating the conversion of glucose to other useful compounds.
Progress in 2021: Sn/SBA15 with Lewis acid point was found to be a good catalyst for the isomerization of glucose to fructose. Sn-Beta with a similar Lewis acid point was shown to be a good catalyst for the decarburization and oxidation of furfural to succinic acid (Fig. 1).
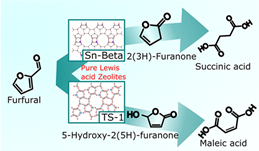
References
- Y. N. Palai, A. Shrotri, A. Fukuoka
Selective Oxidation of Furfural to Succinic Acid over Lewis Acidic Sn-Beta, ACS Catal. 12, 3534-3542 (2022). - P. Chen, A. Shrotri, A. Fukuoka
Unraveling the hydrolysis of β-1,4-glycosidic bond in cello-oligosaccharides over carbon catalysts, Catal. Sci. Technol. 10, 4593-4601 (2020). - P. Chen, A. Shrotri, A. Fukuoka
Soluble Cello-Oligosaccharides Produced by Carbon-Catalyzed Hydrolysis of Cellulose, ChemSusChem 12, 2576-2580 (2019). - A. Shrotri, H. Kobayashi, A. Fukuoka,
Cellulose Depolymerization over Heterogeneous Catalysts, Acc. Chem. Res. 51, 761-768 (2018). - H. Kobayashi, A. Fukuoka
Development of Solid Catalyst-Solid Substrate Reactions for Efficient Utilization of Biomass, Bull. Chem. Soc. Jpn. 91, 29-43 (2018). - H. Kobayashi, H. Kaiki, A. Shrotri, K. Techikawara, A. Fukuoka
Hydrolysis of woody biomass by a biomass-derived reusable heterogeneous catalyst, Chem. Sci. 7, 692-696 (2016). - H. Kobayashi, M. Yabushita, T. Komanoya, K. Hara, I. Fujita, A. Fukuoka
High-Yielding One-Pot Synthesis of Glucose from Cellulose Using Simple Activated Carbons and Trace Hydrochloric Acid, ACS Catal. 3, 581-587 (2013). - A. Fukuoka, P. L. Dhepe
Catalytic Conversion of Cellulose into Sugar Alcohols, Angew. Chem. Int. Ed. 45, 5161-5163 (2006).
2. Depolymerization of chitin and synthesis of organonitrogen compounds
Chitin, which is found in the shells of crustaceans such as crabs and shrimps, is a polymer composed of many N-acetylglucosamine (NAG) chains, and thus, when chitin is used as a renewable resource, the synthesis of characteristic chemicals containing nitrogen is expected. However, chitin is persistent, and the analysis of the organic nitrogen compounds produced by chitin requires a great deal of technique, so research on its degradation and product utilization has been very limited. We have found that acid-impregnated chitin is selectively hydrolyzed to oligosaccharides when treated in a planetary mill.
Progress in 2021: We are interested in how the physical action of milling has an acceleration effect on the catalytic reaction. First, we compared thermal reactions with milling reactions, and found that mechanical forces, rather than local heat generated by the mill, were important. Next, we estimated the force exerted on the chitin molecule by the collision of the milling balls and investigated the chemical effect of the force on the chitin molecule by DFT calculations. The results suggest that not only the tension force, which is intuitively understandable, but also the compressive force activates the glycosidic bond of chitin (Fig. 2).
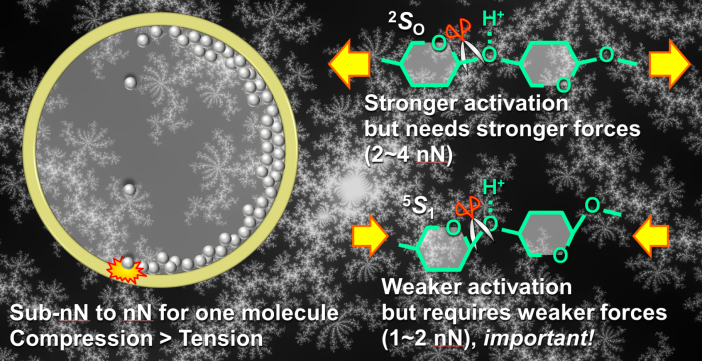
We are interested in how the physical action of milling has an acceleration effect on the catalytic reaction. First, we compared thermal reactions with milling reactions, and found that mechanical forces, rather than local heat generated by the mill, were important. Next, we estimated the force exerted on the chitin molecule by the collision of the milling balls and investigated the chemical effect of the force on the chitin molecule by DFT calculations. The results suggest that not only the tension force, which is intuitively understandable, but also the compressive force activates the glycosidic bond of chitin (Fig. 2).
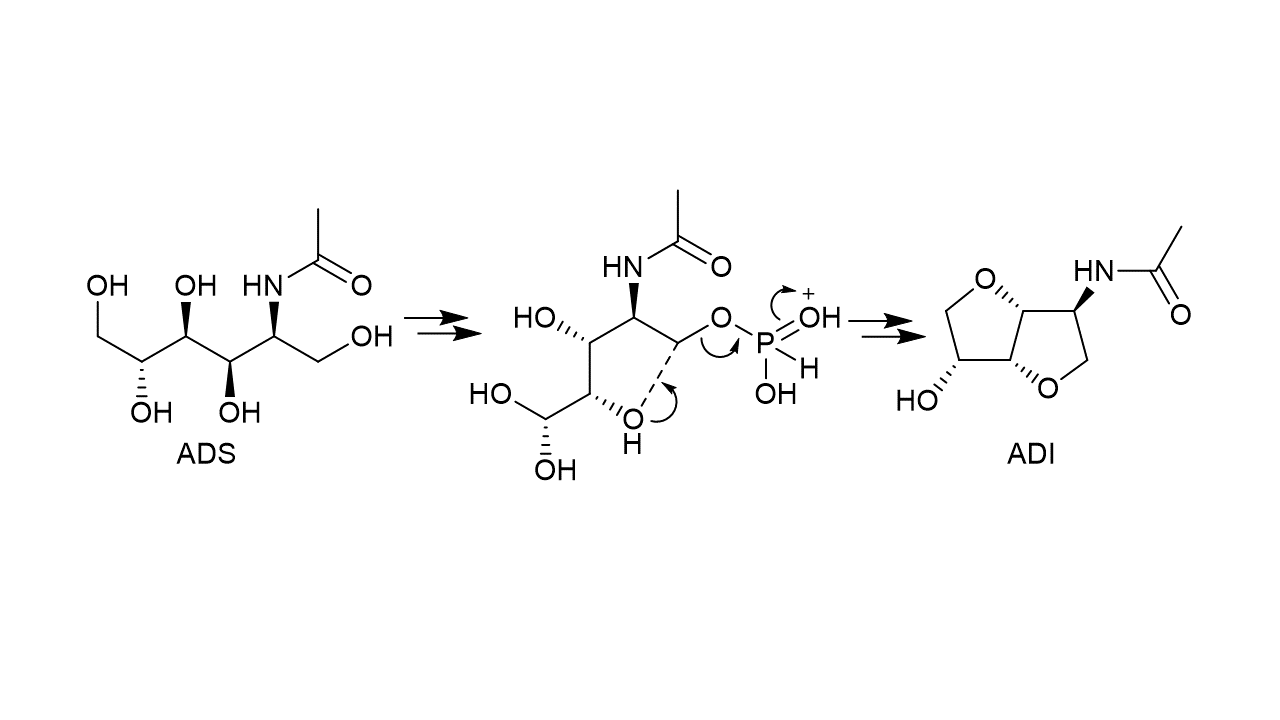
References
- H. Kobayashi, Y. Suzuki, T. Sagawa, K. Kuroki, J. Hasegawa, A. Fukuoka
Impact of tensile and compressive forces on the hydrolysis of cellulose and chitin, Phys. Chem. Chem. Phys. 23, 15908-15916 (2021). - C. Yang, T. Sagawa, A. Fukuoka, H. Kobayashi
Characteristic activity of phosphorous acid in the dehydration condensation of a chitin-derived nitrogen-containing sugar alcohol, Green Chem. 23, 7228-7234 (2021). - D. Padovan, H. Kobayasi, A. Fukuoka
Facile Preparation of 3-Acetamido-5-acetylfuran from N-Acetyl-D-glucosamine Using Commercially Available Aluminum Salts, ChemSusChem 13, 3594-3598 (2020). - T. Sagawa, H. Kobayashi, C. Murata, Y. Shichibu, K. Konishi, A. Fukuoka
Catalytic Conversion of a Chitin-Derived Sugar Alcohol to an Amide-Containing Isosorbide Analog, ACS Sustainable Chem. Eng. 7, 14883-14888 (2019). - K. Techikawara, H. Kobayashi, A. Fukuoka
Acetylglucosamine to Protected Amino Acid over Ru/C Catalyst, ACS Sustainable Chem. Eng. 6, 12411-12418 (2018). - H. Kobayashi, K. Techikawara, A. Fukuoka
Hydrolytic hydrogenation of chitin to amino sugar alcohol, Green Chem. 19, 3350-3356 (2017). - M. Yabushita, H. Kobayashi, K. Kuroki, S. Ito, A. Fukuoka
Catalytic Depolymerization of Chitin with Retention of N-Acetyl Group, ChemSusChem. 8, 3760-3763 (2015).
3. Low temperature oxidation of ethylene by silica-supported Pt catalyst
Ethylene, which is released from various plants such as fruits and vegetables around us, promotes the ripening of fruits, vegetables, and flowers, even though it is only in trace amounts. In particular, there is a great social need to store and transport fruits, vegetables, and flowers while maintaining their freshness, so it is important to develop a technology that can remove ethylene at low temperatures such as room temperature or 0 C. We have found that platinum particles of a few nm in diameter supported on mesoporous silica have an excellent ability to remove ethylene oxidation at low temperatures (Fig. 3). This catalyst can completely remove ethylene at a low concentration of 50 ppm even at 0 C, and was named "Platinum Catalyst" and installed in household refrigerators manufactured by Hitachi GLS, Inc. Furthermore, it was found that the bimetallic catalyst with the addition of ruthenium is also effective in decomposing and removing odor components generated in refrigerators, such as nitrogen-containing or sulfur-containing organic matter. Based on these results, the company decided to install the new "Platinum Catalyst (with ruthenium)" in the vegetable compartment of refrigerators and vacuum chilled refrigerators, and announced the decision in August 2017. The platinum catalyst was used to quantify the catalytic effect on fruit and vegetable freshness retention and to validate the results in storage. This enabled quantification of the rate of ethylene generation from fruits and vegetables and the rate of ethylene degradation by the catalyst. The results showed that when the platinum catalyst was applied to vegetable storage at SECOMA Corporation, the ethylene concentration in the storage decreased and the yield of vegetables increased by approximately 5%, which was effective in reducing food loss.
Progress in 2021: The rate of ethylene generation from fruits and vegetables and the rate of ethylene decomposition by the catalyst were determined, and the amount of catalyst needed could be estimated. Activities of the Food Loss Reduction Consortium, which aims to promote the use of catalyst-based freshness preservation technology, are now in full swing. A "Food Loss Reduction Idea Contest" was held for high school students to promote the use of platinum catalysts, and awards were given to the best schools. These activities were reported in various media such as TV and newspapers (Fig. 4).
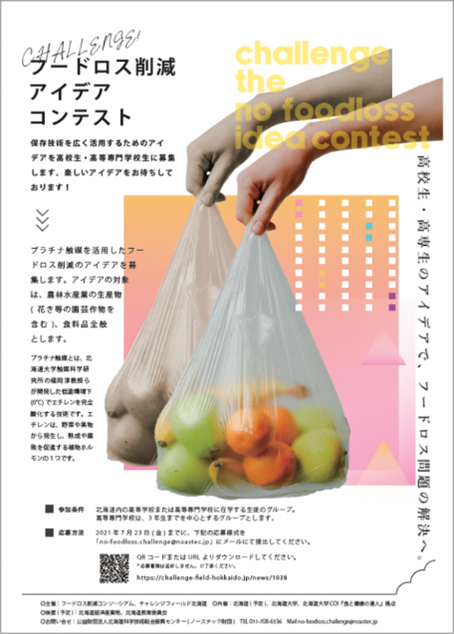
Reference
- T. Mori, T. Shigyo, T. Nomura, Y. Osanai, K. Nakajima, A. Fukuoka
Ethylene oxidation activity of silica-supported platinum catalysts for the preservation of perishables, Catal. Sci. Technol. in press (2022). - S. S. Satter, J. Hirayama, H. Kobayashi, K. Nakajima, A. Fukuoka
Water-Resistant Pt Sites in Hydrophobic Mesopores Effective for Low-Temperature Ethylene Oxidation, ACS Catal. 10, 13257-13268 (2020). - S.S. Satter, J. Hirayama, K. Nakajima, A. Fukuoka
Low temperature oxidation of trace ethylene over Pt nanoparticles supported on hydrophobic mesoporous silica, Chem. Lett. 47, 1000-1002 (2018). - S.S. Satter, T. Yokoya, J. Hirayama, K. Nakajima, A. Fukuoka
Oxidation of Trace Ethylene at 0 °c over Platinum Nanoparticles Supported on Silica, ACS Sustain. Chem. Eng. 6, 11480-11486 (2018). - C. Jiang, K. Hara, A. Fukuoka
Low-Temperature Oxidation of Ethylene over Platinum Nanoparticles Supported on Mesoporous Silica, Angew. Chem. Int. Ed., 52 (24), 6265-6268 (2013).
4. Catalytic conversion of methane
The efficient conversion of methane to syngas (CO + H2) is a key technology for the utilization of natural gas, including shale gas. However, the current industrial steam reforming process (CH4 + H2O → CO + 3H2) is performed at temperatures above 800 C. If methane could instead be converted to syngas by oxidative reforming (CH4 + 1/2O2 → CO + 2H2) at 650 C or lower, energy efficiency would be greatly improved.
Progress in 2021: We have found that Ru-Re/Al2O3 catalysts are highly active in the oxidative reforming of methane. We proposed that the active species is Re with low valence and that Ru plays a role in promoting the reduction of Re. Based on this knowledge, this year we found that the reduction of Re is promoted by using MOR with low Si/Al ratio as a carrier, and that it is active up to a certain level of oxidation atmosphere even without Ru (Fig. 5). The catalyst is expected to stabilize the low valence state by attracting electrons from the Re oxide. The catalyst does not oxidize methane completely, but produces CO and H2O by partial oxidation, followed by H2 production by reforming. Avoiding complete oxidation is an advantage because it mitigates the formation of hot spots.
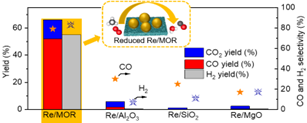
Reference
- L. Li, N. H. MD Dostagir, A. Shrotri, A. Fukuoka, H. Kobayashi
Partial Oxidation of Methane to Syngas via Formate Intermediate Found for a Ruthenium-Rhenium Bimetallic Catalyst, ACS Catal. 11, 3782-3789 (2021). - Y. Hou, S. Nagamatsu, K. Asakura, A. Fukuoka, H. Kobayashi
Trace mono-atomically dispersed rhodium on zeolite-supported cobalt catalyst for the efficient methane oxidation, Commun. Chem. 1, 41 (2018). - Y. Hou, S. Ogasawara, A. Fukuoka, H. Kobayashi
Zeolite-supported rhodium sub-nano cluster catalyst for low-temperature selective oxidation of methane to syngas, Catal. Sci. Technol. 7, 6132-6139 (2017).
5. Catalytic conversion of CO2
Methanol and CO are promising intermediates for the utilization of CO2 as a chemical feedstock via hydrogenation. However, the catalyst systems reported so far have problems such as low product selectivity at high CO2 conversion. To address this issue, we are focusing on the catalytic activity and selectivity of the interface sites of supported metal or metal-doped oxide catalysts. In particular, we are interested in the intrinsic CO2 adsorption and activation ability due to the action of oxygen vacancies and adjacent metal atoms. In this context, we are currently designing various oxide catalysts with oxygen vacancies for selective synthesis of methanol and CO by CO2 hydrogenation.
Progress in 2021: When CO2 hydrogenation (reverse shift reaction) is performed on a catalyst with a single Co atom on ZrO2, CO is produced via formate species with a selectivity of more than 95%. On the other hand, CO hydrogenation is further enhanced in the Co cluster to produce methane. Thus, the selectivity can be changed depending on the size of Co species on ZrO2 (Fig. 6).
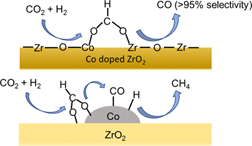
Reference
- N. H. MD Dostagir, R. Rattanawan, M. Gao, J. Ota, J. Hasegawa, K. Asakura, A. Fukuoka, A. Shrotri
Co Single Atoms in ZrO22 with Inherent Oxygen Vacancies for Selective Hydrogenation of CO2 to CO, ACS Catal. 11, 3782-3789 (2021). - N. H. MD. Dostagir, C. B. Thompson, H. Kobayashi, A. M. Karim, A. Fukuoka, A. Shrotri
Rh promoted indium oxide as highly active catalyst for CO2 hydrogenation to methanol, Catal. Sci. Technol. 10, 8196-8202 (2021).